|
10G-EPON
Ethernet Passive Optical Networks
During the past decade, various standards have been developed for realizing PONs. Given that 90 percent of data traffic is in the form of Ethernet frames, it is desirable to have an Ethernet based PON structure in order to reduce the adaptation required for exchanging data between the LAN and the access network.
To this end, IEEE has developed two standards for carrying traffic in the form of Ethernet frames. One is the IEEE 802.3ah Ethernet PON (EPON), also referred to as 1G-EPON standard; the other is 10G-EPON standard developed by IEEE 802.3av task force.
EPON is based on time division multiplexing to avoid collision in the upstream transmission. The data rate is 1 Gbps in both downstream and upstream directions; but the line rates increase to 1.25 Gbps, due to a 25% bit-to-baud overhead incurred by the 8B/10B line encoding. In order to improve bit-error rate and compensate for optical power attenuation, the 1G-EPON standard specifies the Reed-Solomon code (255, 239) as optional Forward Error Correction (FEC) with an electrical gain of 5.9 dB. Generally, the bandwidth allocation procedure is carried out at the OLT, using a medium access control protocol. In the IEEE 802.3ah EPON (1G-EPON) standard, the so-called Multipoint Control Protocol (MPCP) is implemented at the MAC layer for exchanging necessary control information between the OLT and ONUs. MPCP is responsible to perform bandwidth allocation, auto-discovery, and ranging. In MPCP, a GATE message is used by the OLT to convey information to the ONU about the size of the allocated transmission window and the schedule of its transmission and a REPORT message is used by the ONU to transmit information to the OLT about its queue occupancies.
10G-EPON
EPON has been recently extended to 10G-EPON in order to provide ten-fold data rates of 10 Gbps. 10G-EPON has emerged as a promising candidate for the next generation high data rate access systems. This new PON has been standardized under the IEEE 802.3av task force with the aim of developing the physical layer specification and management parameters. The 10G-EPON standard provides symmetric
10 Gbps downstream and upstream, as well as asymmetric 10 Gbps downstream and 1 Gbps upstream data rates. In order to provide backward compatibility with the existing and widely deployed 1G-EPON, the OLT in a 10G-EPON is equipped with dual rate receivers for receiving data from 1G and 10G-ONUs. Furthermore, the downstream transmission channels are separated for sending downstream data and control traffic to 1G- and 10G-ONUs.
The IEEE 802.3av task force focused only on the physical layer and divided it into four sublayers, namely, the reconciliation sublayer (RS), symmetric and asymmetric physical coding sublayer (PCS), physical medium attachment (PMA), and physical medium dependent (PMD) sublayers for symmetric 10 Gbps data rates and asymmetric 10 Gbps downstream and 1 Gbps upstream data rates, while maintaining complete backward compatibility with 1 Gbps EPON equipment. Therefore, the MAC protocol of 1G-EPON remains unchanged. In fact, the 10G-EPON MAC-layer control protocol is an extension of MPCP for 1G-EPON that includes enhancements for management of 10G-EPON FEC and inter-burst overhead.
The 64B/66B line coding in 10G-EPON reduces the bit-to-baud overhead to 3%, compared to the 25% overhead in 1G-EPON which is incurred by 8B/10B line encoding. The burst signal format of 10G-EPON is similar to that of 10G-EPON, except that the receiver settling time of 10G-EPON is twice of that in 1G-EPON, i.e., 800 ns in 10G and 400 ns in 1G-EPON. The laser on/off time and clock data recovery time of both standards are the same (512 ns and 400 ns, respectively).
The wavebands utilized for upstream (US) and downstream (DS) transmissions of 1G- and 10G-EPON standards are illustrated in Fig. 1. As can be seen in this Fig. 1(a), 1G-EPON allocates a 100 nm waveband centered at 1310 nm for upstream transmission and a 20 nm window centered at 1490 nm for downstream transmission. The downstream wavelength of 10G-EPON is allocated in a window between 1575 and 1580 nm (with a typical value of 1577 nm), which is outside of the analog RF video distribution band. Conversely, the upstream wavelength of 10G-EPON is allocated in a 20 nm window centered at 1270 nm which is completely covered by a part of the 1G-EPON upstream waveband.
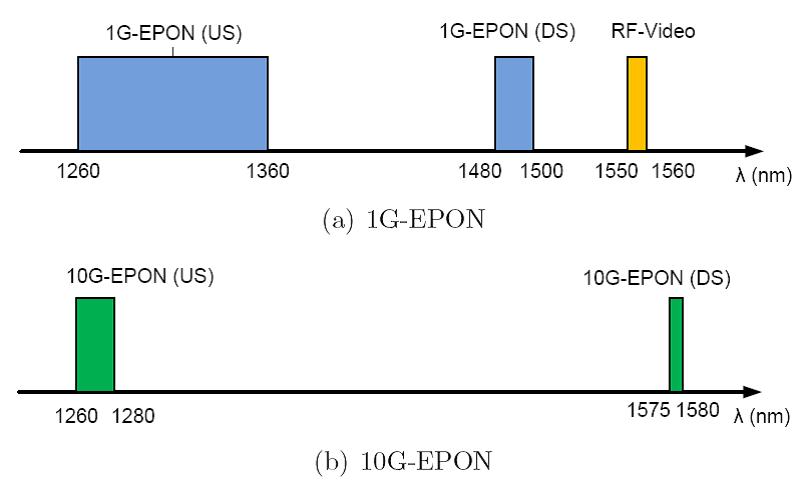
Fig. 1. Waveband allocation in 1G- and 10G-EPON.
Unlike 1G-EPON, implementation of a FEC code is mandatory in 10G-EPON in order to realize its new power budget class. Considering several aspects of various FEC codes, such as the gain, circuit size, and latency associated with encoding and decoding, The Reed-Solomon code (255, 223), which is a linear cyclic block code, was adopted for 10G-EPON to enhance the FEC gain and alleviate optical transceiver specifications. This FEC has an electrical gain of 7.2dB, which allows lower power signal detection with the same bit error rate compared to the optional FEC utilized in legacy 1G-EPONs. However, the FEC in 10G-EPON results in up to 12.9% overhead which is the major overhead component in 10G-EPON.
The backward compatibility requirement of the new and existing EPONs introduces several technical challenges and difficulties on the specification work such as a high power budget exceeding 30 dB for symmetric 10 Gb/s transmission, conflicts in wavelength allocation, and dual-rate burst-mode operation at the OLT receiver. Two main techniques are employed for achieving the coexistence of 10G-EPON with 1G-EPON (and analog RF video distribution) systems: WDM overlay in the downstream direction and a dual-rate burst-mode receiver in the upstream direction to support a dual-speed TDM.
In the downstream direction, since the wavelength bands are distinct, a WDM overlay is a straightforward way to provide the coexistence with the legacy 1G-EPON. On the contrary, as depicted in Fig. 1, the upstream wavelength band of 10G-EPON is in fact a subset of the 1G-EPON waveband; hence a dual-rate burst-mode operation is the only remaining option to retain the coexistence requirement by using dual-speed TDM. For this purpose, the OLT is equipped with dual-rate mode receiver. Moreover, the OLT provides three kinds of MAC instances for operating on symmetric and asymmetric data rates; namely, the OLT supports 1/1 Gb/s, 10/1 Gb/s, and 10/10 Gb/s MAC instances. A typical network architecture for the co-existence of 1G- and 10G-EPONs is illustrated in Fig. 2.
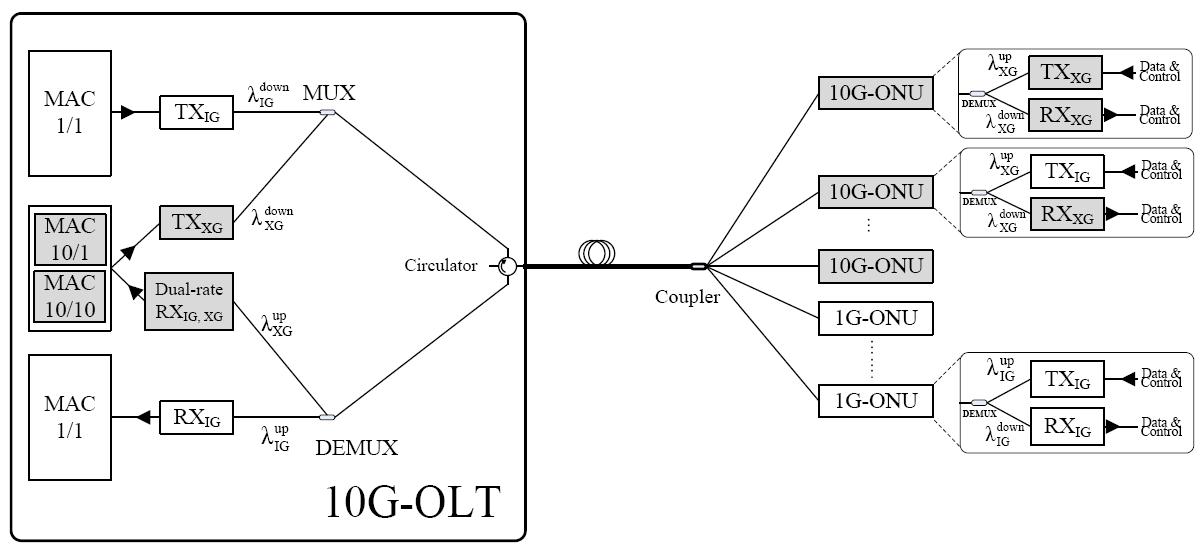
Fig. 2. A typical network architecture for the co-existence of 1G- and 10G-EPONs.
Further reading
- K. Tanaka, A. Agata, and Y. Horiuchi,
“IEEE 802.3av 10G-EPON standardization and its research and development status,”
IEEE/OSA Journal of Lightwave Technology, vol. 28, no. 4, pp. 651-661, Feb. 2010.
- G. Kramer,
“10G-EPON: Drivers, challenges, and solutions,”
in Proc., European Conference on Optical Communication (ECOC '09), Vienna, Austria, Sept. 2009, pp. 1-3.
- M. Hajduczenia, H. J. A. D. Silva, and P. Monteiro,
“Development of 10 Gb/s EPON in IEEE 802.3av,"
IEEE Communications Magazine, vol. 46, no. 7, pp. 40-47, July 2008.
|
|
|
| |